Abstract
The
Zero Energy Manufactured Home Project demonstrates and promotes
innovative energy saving technologies to the manufactured housing
industry and home buying public, while evaluating those technologies’ energy
performance. The project, funded by the Bonneville Power Administration,
and the U.S. Department of Energy’s (DOE) Building America
Industrialized Housing Program (BAIHP), examines two 147 square
meter (m 2) (1600 ft 2) two-section manufactured homes, built
by the same manufacturer, using an identical floor plan. Heating
ventilation and air-conditioning (HVAC) measurements, envelope
and duct leakage tests were conducted and remote monitoring
equipment installed to track the performance of each home over
a three year period.
Both
homes were built in the summer of 2002 and sited in the cold,
dry climate of Lewiston, ID. The Zero Energy Manufactured Home
(ZEMH) has been built with highly efficient and cutting edge
technologies including a photovoltaic system, sun-tempering,
solar water heating, spray-in foam insulation, heat recovery
ventilation, and Energy Star appliances, HVAC and lighting The
comparison (ESTAR) home is built to Energy Star Program requirements
as part of the Northwest Energy Efficient Manufactured Home program,
which includes a quiet 20 watt whole house exhaust fan ventilation
system. Energy Star is the most energy efficient home that meets
the U.S. Department of Housing and Urban Development code available
in the United States market today (NEEM).
This
paper presents the field testing and monitoring results of both
homes’ heat recovery (HRV) and non-heat recovery (NHRV)
ventilation system energy performance, based on in-situ monitoring
data. Energy Gauge USA computer simulation software is used to
compare the ventilation, envelope and duct leakage induced infiltration
in the ZEMH and ESTAR homes. Anecdotal and occupant related observations
are presented, along with some conclusions.
Keywords
Heat
recovery ventilation, indoor air quality, energy efficiency
Poster
View
the one-page poster of this paper. Poster (pdf
- 417kb)
Introduction
More
than 200,000 factory homes are built in the United States each
year to the federal manufactured housing standards (HUD 1994).
Issues related to energy efficiency, heating, ventilation and
air-conditioning standards and performance in these homes has
been presented in previous AIVC papers (Lubliner 2000, 2003 ),
(Persily 2003).
HUD
code manufactured homes tend to be built tighter than site built
homes, which is why codes require whole house mechanical ventilation
systems. (HUD 1994), (TenWolde 1996), (Stevens 1997). HUD requires
ventilation systems capable of ventilating the ZEMH and ESTAR
homes be at least 26 liters per second (l/s) (56 ft 3/min). For
these homes the sizing approach in the American Society of Heating,
Refrigerating and Air-Conditioning Engineers (ASHRAE) Standard
62 (ASHRAE 2003) would require a comparable 29 l/s (61 ft 3/min).
The
ZEMH was built with energy efficiency and renewable energy as
a high priority, in an effort to evaluate proposed future energy
efficiency targets. The BAIHP targets seek to demonstrate future
whole house energy savings of 60-70 percent over current practice,
while improving indoor air quality and durability. (Lubliner
1994) Energy modeling of the ZEMH suggests that it achieves the
60 percent target. The ESTAR home achieved the current BAIHP
30 percent target. Analysis that compares modeled and actual
total and end-load technology energy use in ZEMH and ESTAR home
is currently under way.
HVAC
System Descriptions:
Heat
Pump: Both the ZEMH and ESTAR homes are all-electric homes
with HVAC systems located in the utility room. They both have
7 kilowatt (kW) (2 ton) ducted unitary air-to-air heat pumps
with electric resistance backup. All heat pump components,
including the outdoor coil, are located inside a small closet
in the house. The heat pump draws air from the crawlspace,
directs it across the outdoor coil, and exhausts it through
the ceiling and roof cap. Investigations are currently under
way to determine the heating and cooling performance of the
heat pump compared with typical split system heat pumps and
electric furnaces.
Ductwork: The
heat pump duct system has a single return air grill located in
the utility room. Supply ducts distribute conditioned air to
floor registers in the other rooms via riser and trunk ductwork
located above the floor insulation. A crossover duct in
the vented crawlspace connects the trunk ducts of the two home
sections. The use of duct mastic and air-tight Icynene ™ foam
floor insulation in the ZEMH ensures that the supply riser and
trunk ducts are airtight and well within the indoor pressure
and insulation boundary with minimal heat transfer between the
house and the crawlspace. The ESTAR home has a leakier duct system
as a result of the use of foil duct tape and because the ducts
are located in a loose-fill insulation. Since 2003 all Energy
Star homes in the Pacific Northwest are required to use mastic
instead of tape to seal ductwork. The forced air distribution
system introduces inefficiencies caused by conduction heat transfer
between the ducts and the crawlspace, duct leakage to the crawlspace,
and duct leakage induced infiltration. Duct leakage induced infiltration
results when supply air duct leakage causes negative pressures
within the home relative to the outdoors. Supply duct leakage
has been shown to be a significant contributor to uncontrolled
air infiltration that increases with the HVAC operational time.
(Palmiter 1992) (Persily 2000, 2003). During the winter heating
season, longer HVAC operation time combined with greater stack
infiltration result in high air change rates. In homes with leaky
ducts and envelopes this can result in significant over ventilation
and reduces the need for the continuous mechanical ventilation
system to operate. The ZEMH’s tighter ducts and building
envelope reduce winter periods of over ventilation making
the HRV an important component to the philosophy “build
tight; ventilate right.”
Table
1: Duct & Envelope leakage and Ventilation System Flow
Rates
Test |
ZEMH |
ESTAR |
Envelope
Leakage1 |
2.0
ACH @ 50Pa |
3.6
ACH @ 50Pa |
Total
Duct Leakage2 |
145
CFM @ 25Pa
(68 L/s @ 25Pa)
15% of HVAC flow |
211
CFM @ 25Pa
(100 L/s @ 25Pa)
20% of HVAC flow |
Duct
Leakage to Outside2 |
37
CFM @ 25Pa
(17 L/s @ 25Pa)
4% of HVAC flow |
150
CFM @ 25Pa
(71 L/s @ 25Pa)
15% of HVAC flow |
Whole
House Ventilation |
70
CFM (33 L/s)3 |
78
CFM (37 L/s) |
1
Tested per ASTM Standard E779-87
2 Measured per ASHRAE Standard 152-2002
3 Measured on low-speed with clean pre filter and 3 month
old HEPA filter |
Table
1 provides a comparison of supply duct and envelope leakage in
ZEMH and ESTAR homes using Duct Blasters™ and Blower Doors™ typically
employed to test energy efficient homes. Both the ductwork and
envelope are considerably tighter in the ZEMH. The difference
in both total duct leakage and duct leakage to outside in the
ZEMH show the ducts are tighter and that more air leaked through
the ducts goes back into the house instead of the crawlspace.
HRV
Operation: HRVs are typically used in cold climates to
efficiently supply a steady flow of fresh outdoor air. As stale
warm air is expelled, the heat recovery core warms the incoming
fresh, colder air before it is distributed throughout the home.
The result is a constant supply of fresh air, no unpleasant
drafts, and greater home comfort. In addition to heat recovery
and improved air quality, the HRV provides necessary ventilation
while controlling excess humidity. The HRV in the ZEMH is a
Venmar 3000™, which is designed to save energy while
ventilating and providing High Efficiency Particulate Air Filters
(HEPA) filtration of outside and re-circulated air. The Venmar
3000 has a replaceable HEPA filter and separate pre-filter.
The ZEMH HRV measured flow rates were 33-42 l/s (70-90 ft 3/min)
of fresh outside air and exhausts a comparable amount
of stale indoor air. The operation of the balanced HVR
does not cause house depressurization and associated air leakage.
Stale exhaust air is drawn through the heat exchanger core. The
occupant controls include a normal mode for continuous operation
33 l/s (70 ft 3/min) and a higher boost mode that can
be used by occupants when higher levels of indoor air pollutants
are present 42 l/s (90 ft 3/min). The occupant controls also include
a re-circulation mode where HEPA filtration occurs with no air
exhausted or introduced and hence no ventilation. The Venmar HEPA
3000 promotes filtration and associated improved indoor air quality
(IAQ), unlike many HRV systems specifically designed solely for
heat recovery. The ZEMH HRV provides 66 l/s (140 ft 3/min ), of
re-circulated HEPA filtered air and mixes it with a measured 33
l/s (70 ft 3/min) of pre-heated outdoor air for a total of 104
l/s (220 ft 3/min) supplied to the home on normal speed. The 104
l/s (220 ft 3/min) delivered from the HRV is ducted 9 meters (30
ft) using 20 centimeter (8 in) diameter flexible plastic duct located
under the attic foam insulation to a ceiling grill in the bedroom
hallway at the other end of the home from the HRV return.
Defrost
Mode: To ensure that the HRV does not freeze the HEPA 3000
employs a defrost mode with variable timing depending on the
outside temperature. When the outside air temperature is between
-5º C and -15ºC (23ºF and 5ºF), a mechanical
damper shuts the outside air entering the HRV for six minutes
every 30 minutes as the defrost mode. This allows the re-circulation
air to warm the core. When the outside temperature is between
-15ºC and -27ºC (5ºF and -17ºF), the HRV
runs in defrost mode for nine minutes every 30 minutes, and
20 minutes every 30 minutes when the outside temperature is
below -27º C (-17ºF). (Forest) During 2003-2004,
the HEPA 3000 was in defrost mode for about 9 hours.
HRV
Distribution Effectiveness: The HRV duct design in the
ZEMH is independent of the heat pump ducts and not directly
ducted to bedrooms. Connecting the HRV to the heat pump ducts
would require the 350 watt heat pump blower fan to operate
continuously, causing electricity use of up to 3300 kilowatt-hours
(kWh) per year. The ZEMH independent duct design reduces energy
use. However, it also reduces spatial mechanical ventilation
effectiveness in bedrooms when the heat pump is not operating.
Research has shown that spatial ventilation effectiveness is
improved as the central forced air heating/cooling system operates
more frequently to mix the fresh air from the HRV with the
indoor air. (Persily 2000)
Figure
2. Run time of the ZEMH and ESTAR homes vs. Outside Temperature
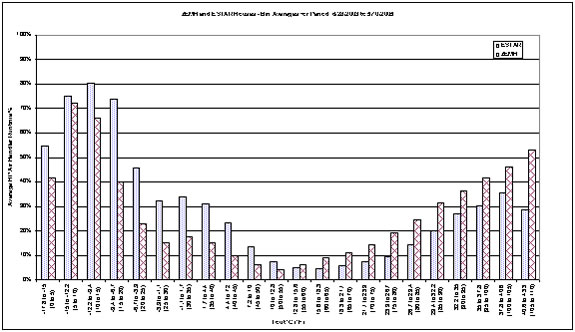
Figure
2 shows the percentage runtime as a function of outdoor temperature
for both the ZEMH and ESTAR home. As expected the runtime increases
as the outdoor temperature gets lower during the heating season
and increases as the outdoor temperature get higher during the
air-conditioning season. The lower runtime in the ZEMH during
heating is because the home is more energy efficient and both
homes use identical heat pumps. The higher runtime in the ZEMH
during the cooling season is a result of the occupant using the
heat pump in air-conditioning mode more often in part due to
dust, simplicity, and daytime occupancy. The ESTAR occupant relies
more on opening windows at night to provide diurnal cooling,
instead of using the heat pump. Both occupants will typically
open windows when the outside temperature falls within this temperature
range. When the heat pump system runs at night, occupants benefit
from greater air distribution to the bedrooms. A Venmar duct
accessory product currently under development will integrate
both duct systems and allow for HRV fresh air to be supplied
to all ducted rooms without requiring the operation of the higher
wattage heating/cooling fan. This product will improve spatial
ventilation effectiveness, will have no negative impact on heating
and cooling systems if it uses the same ductwork, and will reduce
fan energy use by not relying on the operation of the air handler
blower. Another innovative ventilation control used to improve
ventilation effectiveness in many energy efficient site built
homes is the Fan Recycler™, which ensures that ventilation
and indoor air are mixed by the existing forced air system a
minimum set time period every hour, by monitoring the HVAC fan
runtime. (Rudd)
HRV
Maintenance and Operation: The HEPA filter is recommended
to be replaced every year. The pre-filter of the HEPA cartridge,
HRV core filters and inside of the HRV should be cleaned every
six months. The difference in flow rates between the clean
and dirty pre-filter was found to be less than 10%, with the
HVR core 6-months old. The occupants were asked to run the
HRV continuously and use the boost mode when they desired additional
ventilation. The HRV in the ZEMH was operated primarily in
normal mode. The boost mode was rarely used. The re-circulation
mode eliminates outside air. This mode is intended for use
during periods when outdoor pollutants are present. Instead
of using the re-circulation mode, the occupants shut the HRV
off because of problems with dust, insects and barbeque smoke,
which they associated with the HRV. These issues resulted in
the HRV being turned off for extended periods of time during
the summer of 2003. Discussions with occupants and resolution
of problems resulted in HRV continuous operation in 2004-05.
HRV
Efficiency: The tested HRV net efficiency is 56%
provided by Venmar for the HEPA 3000. The net efficiency varies
with outside air temperature and flow rate. While there is
no industry approved method or test to evaluate the energy
recovery efficiency of multifunction products such as the Venmar
HEPA 3000, laboratory testing was conducted using CSA C439-00
C439-00 (Standard Laboratory Methods of Test for Rating the
Performance of Heat/Energy-Recovery Ventilators) with some
adaptations to take into account the impact of blending indoor
air inside the unit. The net efficiency has been evaluated
from the gross temperature recovery efficiency (defined as
apparent effectiveness in CSA C439) to which several penalties
were subtracted: unbalance factor, exhaust air transfer, casing
air leakage, casing heat gain/losses, defrost energy (when
applicable) and fan energy. (Forest)
The
measured power consumption of the HRV blower motor in the ZEMH
was 164 watts and 228 watts on normal mode and boost mode, respectively.
The manufacturer reports that 85 percent of the heat generated
from the motor is transferred to the incoming air downstream
of the heat exchanger core with the remaining 15 percent transferred
to the exhaust air downstream of the core. The high wattage of
the Venmar 3000 blower is a result of the HEPA filtration option.
Other small wattage Venmar HRV models such as the Duo 1.2™ do
not employ HEPA filters and uses only 68 watts to provide 32
l/s (68 ft 3/min) at an 87 percent apparent sensible effectiveness
at 0º C, (32º F).
NHRV: The
ESTAR home utilized a Panasonic #FV-08Q2 Whisper Ceiling™ whole
house exhaust fan. The fan is located in the utility room to
provide non-heat recovery ventilation. The fan is designed and
controlled to operate continuously. A circuit breaker must be
turned off to disable operation. The exhaust flow rate was measured
at 37 l/s (78 ft 3/min) using a flow grid that measures average
velocity. Unlike the HRV, the NHRV fan causes a slight depressurization
of the home relative to the outdoors. This depressurization causes
outside air to enter the home via air leakage pathways in the
thermal envelope and ductwork. The location of these leakage
pathways dictates the spatial ventilation effectiveness associated
with the NHRV system when the heat pump is not operating. Depressurization
from the supply duct leakage and NHRV increases ventilation rates.
The NHRV exhaust fan flow rate is added in quadrature to the
stack effect because of changes in the vertical neutral pressure
location. This results in higher flow rate capacity fan required
to provide equal mechanical ventilation as the balanced HRV system.
For the balanced HRV, the flow rate and stack effect are simply
additive.
Energy
Gauge USA Simulation: Energy Gauge USA version 2.4 was
used to estimate the space heating, cooling and ventilation
fan energy for the ZEMH and ESTAR homes as shown in Table 2a.
Table 2b compares energy use for a variety of real and theoretical
cases. All ventilation cases assume 33 l/s (70 ft 3/min). The
difference between runs No.1 to No.2 in ZEMH and No.5 to No.6
in ESTAR show the energy savings associated with not using
the HEPA option when using an HRV. The difference between runs
No.2 to No.3 in the ZEMH and No.6 to No.7 in ESTAR show the
energy savings associated with the HRV over the NHRV. The difference
between the Non-ventilation Case 8 over the other ventilation
cases highlights the cost of ventilation to achieve improved
IAQ. The ventilation system case comparisons in Table 2b indicate
greater savings for the ESTAR home than the ZEMH. This is believed
to be a result of ZEMH having both lower house and heat pump
balance points.
Table
2A: Energy Gauge Simulation Energy Use Results
Case |
Home |
Type |
Efficiency |
Watts |
Heat
kWh/yr |
Cool
kWh/yr |
Heat
+ Cool |
1 |
ZEMH |
HEPA |
56% |
164 |
7124 |
286 |
7410 |
2 |
ZEMH |
DUO |
87% |
70 |
5759 |
236 |
5995 |
3 |
ZEMH |
NHRV |
0% |
20 |
7730 |
256 |
7986 |
4 |
ZEMH |
NONE |
0% |
0 |
5045 |
204 |
5249 |
5 |
ESTAR |
HEPA |
56% |
164 |
8523 |
455 |
8978 |
6 |
ESTAR |
DUO |
87% |
70 |
7034 |
394 |
7428 |
7 |
ESTAR |
NHRV |
0% |
20 |
9310 |
424 |
9734 |
8 |
ESTAR |
NONE |
0% |
0 |
6268 |
358 |
6626 |
Table
2B: Case Comparisons
HEPA
filtration Cost: |
kWh/yr |
HEPA
vs. Duo in ZEMH |
1415 |
HEPA
vs. Duo in ESTAR |
1550 |
HRV
Savings vs. NHRV: |
kWh/yr |
Duo
vs. NHRV in ZEMH |
1991 |
Duo
vs. NHRV in ESTAR |
2306 |
Cost
of Vent vs. None: |
kWh/yr |
HEPA
vs. None in ZEMH |
2161 |
HEPA
vs. None in ESTAR |
2352 |
Duo
vs. None in ZEMH |
746 |
Duo
vs. None in ESTAR |
802 |
NHRV
vs. None in ZEHH |
2737 |
NHRV
vs. None in ESTAR |
3108 |
Costs: The
HEPA 3000 costs $1,100 U.S., Duo 2 costs $700 U.S. and the NHRV
costs $150 U.S. These are costs of the equipment and do not reflect
installation, and markups. The HEPA 3000 has a $100 U.S. annual
maintenance cost to replace the HEPA filter. The other units
have no annual maintenance costs; cleaning maintenance is assumed
to be provided by the occupants at no cost. The useful life of
these systems is unknown. It should also be noted that the HEPA
filtration non-energy benefits to indoor air quality need to
be considered when looking at first and operating costs. These
benefits may include the avoidance of health care expenses—such
as the cost of a respiratory inhaler and doctor visits—which
can far outweigh the cost of energy recovery.
Conclusions
- HRV
systems can improve energy efficiency in new U.S. manufactured
housing especially in homes with tighter duct and envelope
systems.
- Low
fan energy is a factor in HRV and NHRV systems energy performance.
- HRV
systems with HEPA filtration require additional fan energy,
but provide non-energy related IAQ filtration benefits.
- HRV
and NHRV continuous operating systems provide effective ventilation
IAQ filtration benefits with varying associated energy costs.
- Controls
that reduce over-ventilation are needed especially in homes
with leakier ductwork and envelopes (i.e. many non-ESTAR homes).
- Innovative
controls and duct components that integrate heating/cooling
ductwork with the HRV may improve spatial and temporal ventilation
effectiveness.
- Cost
analysis alone may not provide “apples-to-apples” comparisons
in terms of IAQ benefits.
Acknowledgments
This
work is sponsored in large part by the U.S. Department of Energy
Office of Building Technology’s Building America Industrialized
Housing program under Cooperative Agreement DE-FC36-99GO10478.
Special thanks to Daniel Forest, Venmar Ventilation Inc. Additional
support has been provided by: The Bonneville Power Administration,
the Florida Solar Energy Center, the Washington State University
Extension Energy Program, the Nez Perce Tribe, Kit Manufacturing,
Clearwater Homes and the Northwest Energy Efficient Manufactured
Home Program.
References
American
Society of Heating, Refrigerating and Air-Conditioning Engineers
(1988) ASHRAE Standard 119-1988, Air Leakage Performance for
Detached Single-Family Residential Buildings.
American
Society of Heating, Refrigerating and Air-Conditioning Engineers
(2003) ANSI/ASHRAE Standard 62.2, Ventilation for acceptable
indoor air quality.
Canadian
Standards Association (2000) CSA C439-00. Standard Laboratory
Method Test for Rating the Performance of Heat/Energy Recovery
Ventilators.
ECOTOPE
2003 Summary of SGC Manufactured Home Field Data 1997-98: Sitings
in Idaho and Washington. Ectope, Inc., Seattle, WA.
Forest,
D. Conversations with Danial Forest, Venmar AVS, 2005.
U.S.
Department of Housing and Urban Development (1994) Manufactured
Home Construction and Safety Standards, Part 3280. Washington,
D.C.
Lubliner,
M.; Stevens, D.T.; and Davis, B. (1997) Mechanical ventilation
in HUD-code manufactured housing in the Pacific Northwest. ASHRAE
Transactions, 103 (1), pp. 693-705.
Lubliner,
M. et al (2003) Building Envelope, Duct Leakage and HVAC System
Performance in HUD-code Manufactured Homes. 24 th AIVC Conference
Proceedings. Brussels, Belgium.
Lubliner,
M. et al (1997) Mechanical ventilation in HUD-code manufactured
housing in the Pacific Northwest. ASHRAE Transactions,
103 (1), pp. 693-705.
Lubliner,
M.; Gordon, A. (2000) Ventilation in U.S. Manufactured Homes:
Requirements, Issues and Recommendations. 21 st AIVC Conference
Proceedings.
Lubliner,
M, et al (2004) Manufactured Home Performance Case Study: A Preliminary
Comparison of ZeroEnergy and Energy Star. Performance of Exterior
Envelopes of the Whole Building IX International Conference,
American Society of Heating, Refrigerating and Air-Conditioning
Engineers.
NEEM
(2004) Northwest Energy Efficient Manufactured Home Program In-Plant
Inspection Manual, Oregon Office of Energy.
Palmiter,
et al (1992) Measured Infiltration and Ventilation in Manufactured
Homes. Ecotope, Inc.
Persily,
A.K. (2000) A Modeling Study of Ventilation in Manufactured Homes.
National Institute of Standards and Technology, Gaithersburg.
Persily,
A.K., et al (2003) Ventilation Characterization of a New Manufactured
Home. 24 th AIVC Conference Proceedings. Air Infiltration
and Ventilation Centre, Brussels, Belgium.
Rudd,
Armin F.; Lstiburek , Joseph W. (2000) Measurement of Ventilation
and Interzonal Distribution in Single-Family Homes. ASHRAE
Transactions 2000, MN-00-10-3, V. 106, Pt.2., American Society
of Heating Refrigeration and Air-Conditioning Engineers, Atlanta,
GA.
Stevens,
D.T; Lubliner, M; Davis, B. (1997) Mechanical ventilation in
HUD-code manufactured housing in the Pacific Northwest. ASHRAE
Transactions, 103 (1), pp. 693-705.
TenWolde,
A.; Burch, D.M. (1996) Ventilation, Moisture Control, and Indoor
Air Quality in Manufactured Houses. Forest Products Laboratory,
National Institute of Standards and Technology. |